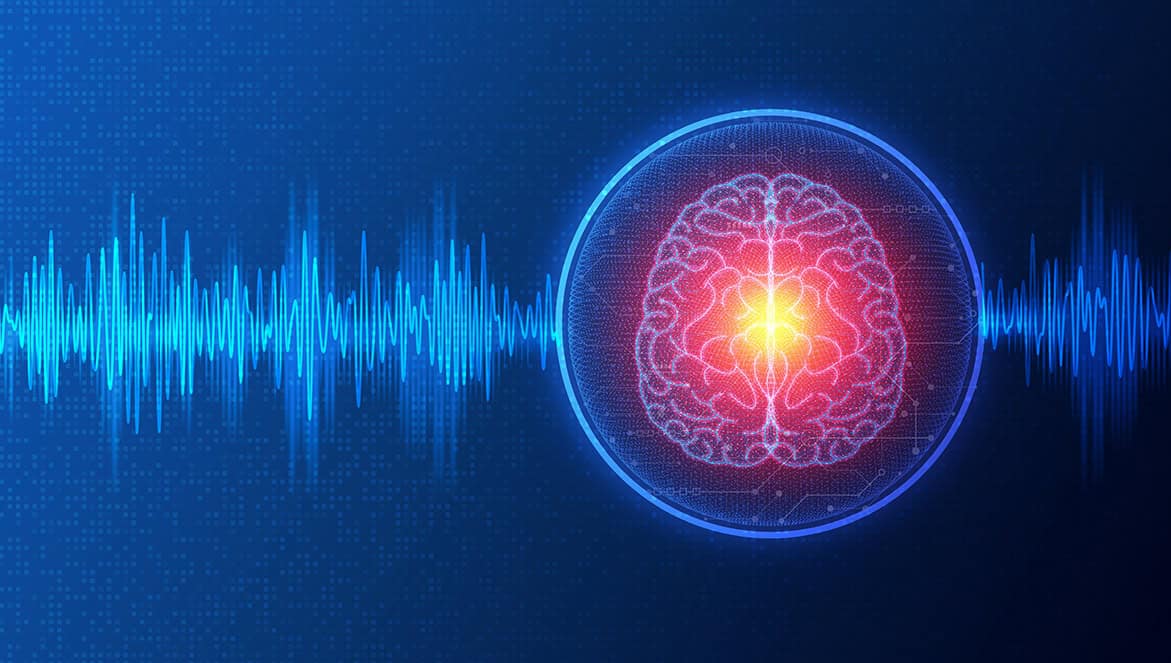
By Catherine Steffel 16 May 2024
Collected at : https://physicsworld.com/a/next-generation-quantum-sensors-detect-human-biomagnetism
Anna Kowalczyk, an assistant professor in the University of Birmingham’s Centre for Human Brain Health, has set out to develop new tools that could help neuroscientists do their work better.
“Our motivation is to use transcranial magnetic stimulation with a brain imaging technique called magnetoencephalography, and this is a very challenging task, because commercial sensors may not work well in this setup,” Kowalczyk says. “I wanted to start developing quantum sensors for brain imaging, and I basically started from scratch.”
Transcranial magnetic stimulation uses magnetic fields to stimulate neurons in the brain by placing a magnetic coil against the scalp and generating brief magnetic pulses that induce electrical currents in the brain. Magnetoencephalography measures the magnetic fields produced by this resultant activity and can be used to map brain activity and understand the relationships between brain regions and their functions.
Optically pumped magnetometers (OPMs) are emerging as preferred sensors for biomagnetism applications. Commercial OPMs used for magnetoencephalography are “zero field sensors,” meaning that they can detect very small magnetic fields, often at femtotesla-level sensitivities. Many zero-field sensors are sensitive to environmental magnetic fields and so require extensive shielding systems and noise cancellation techniques.
Kowalczyk’s research, led by Harry Cook, a graduate student studying physics at the University of Birmingham, decided to employ “slightly different physics” with their sensors. “We decided to make use of slightly different physics to develop a sensor that can work in higher magnetic fields with great precision,” says Kowalczyk.
Their prototype sensor is an optically pumped magnetic gradiometer (OPMG) that operates by nonlinear magneto-optical rotation: linearly polarized light passes through a rubidium vapour, preparing the atoms in the vapour to be magnetically sensitive. When an external magnetic field changes, the frequency of atoms’ precession around the magnetic field changes, which is in turn detected with laser light. In other words, changes in the magnetic field are tracked by measuring changes in light properties.
With one “cell” of rubidium vapour, the researchers can measure an external magnetic field locally. With two cells, they can directly measure the difference, or gradient, in the magnetic field (i.e., their sensor becomes a gradiometer).
After designing and prototyping the sensor, the researchers tested its performance by characterizing an auditory evoked response in the human brain, a common benchmark for OPMs. Next, they set out to measure the field from a human heartbeat. During recording, the building lifts were running and the team recorded the resulting magnetic disturbance using commercial sensors.
“[In our experimental setup] we’re inside this metal box, basically, but the [external field] is still many times larger than a human heartbeat, let alone the human brain. So we wanted to see how well our sensor could measure the gradient from the heart while rejecting the unwanted magnetic field,” Cook explains. “It wasn’t a perfect setup, but we could clearly see that there’s a human heartbeat that’s well-characterized from a magnetic perspective…Our gradient method remained almost flat during this measurement, while the reference sensor showed all the variations.”READ MOREQuantum physics gives brain-sensing MEG scanners a boost
The researchers concluded that the OPMG could be explored further for biomagnetism applications and are pursuing two directions for research going forward: improving the current sensor; and developing sensors that could measure multiple types of neural activity simultaneously.
“We need to make the sensor more robust…and we need to make more sensors. The main motivation is to make it work with transcranial magnetic stimulation, and we also want to make hybridized quantum sensors that can measure two kinds of neural activities at the same time,” says Kowalczyk, referencing functional near infrared spectroscopy, another optical method for measuring brain activity. “Each method would bring different kinds of information. We’re not saying that our sensor is better than commercial sensors, it’s just different. Our aim is to develop technology that enables new approaches and new capabilities in neuroscience and beyond.”
Leave a Reply