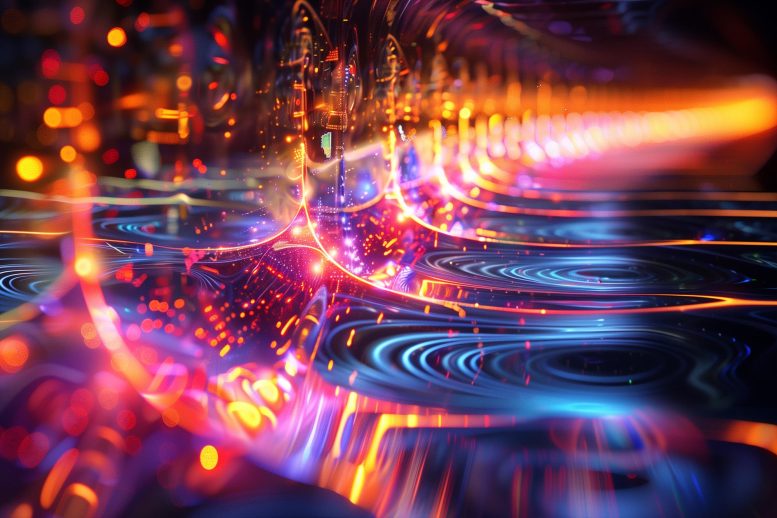
By Rice University December 20, 2024
Collected at: https://scitechdaily.com/quantum-spin-liquids-are-real-and-could-change-technology-forever/
Scientists have found evidence of a strange state of matter called a quantum spin liquid in a material known as pyrochlore cerium stannate.
In this mysterious state, magnetic particles don’t settle into a fixed pattern but stay in constant motion, even at extremely low temperatures. Researchers used advanced tools like neutron scattering and theoretical models to detect unusual magnetic behavior that behaves like waves of light. This breakthrough could lead to new discoveries in physics and future technologies like quantum computing.
Quantum Spin Liquids
Physicists have long speculated about the existence of a rare state of matter called a quantum spin liquid. In this state, magnetic particles refuse to settle into an orderly arrangement, even at absolute zero temperature. Instead, they stay in a constantly shifting, entangled state driven by the strange rules of quantum mechanics. This creates unusual properties that resemble fundamental interactions in the universe, such as the way light and matter behave. Despite its potential significance, proving the existence of quantum spin liquids through experiments has been notoriously difficult.
Groundbreaking Discovery
In a recent study published in Nature Physics, an international team of researchers, including experimental physicists from Switzerland and France and theoretical experts from Canada and the U.S., uncovered evidence of this mysterious quantum state in a material called pyrochlore cerium stannate. They combined cutting-edge experimental techniques, such as neutron scattering at ultra-low temperatures, with advanced theoretical analysis. By examining how neutrons interact magnetically with the electrons’ spins in pyrochlore, they detected collective spin excitations behaving like lightlike waves, supporting the presence of a quantum spin liquid.
Advancements in Neutron Scattering
“Fractional matter quasiparticles, long theorized in quantum spin liquids, required significant advancements in experimental resolution to be convincingly tested in this type of material,” said Romain Sibille, the leader of the experimental team at Paul Scherrer Institute in Switzerland. “The actual neutron scattering experiment was performed on a highly specialized spectrometer at the Institut Laue-Langevin in Grenoble, France, allowing us to obtain extremely high-resolution data.”
“Neutron scattering is a well-established tool in analyzing the behavior of spins in magnets,” added Andriy Nevidomskyy, associate professor of physics and astronomy at Rice who conducted theoretical analysis of the acquired data. “It is very difficult, however, to come up with an unambiguous ‘smoking gun’ signature that would prove the material harbors a quantum spin liquid.”
Indeed, a 2022 study by Nevidomskyy showed that narrowing the theoretical model to dependably describe the experiment is far from easy, requiring numerically sleuthing out the model parameters and fitting it to multiple experiments.
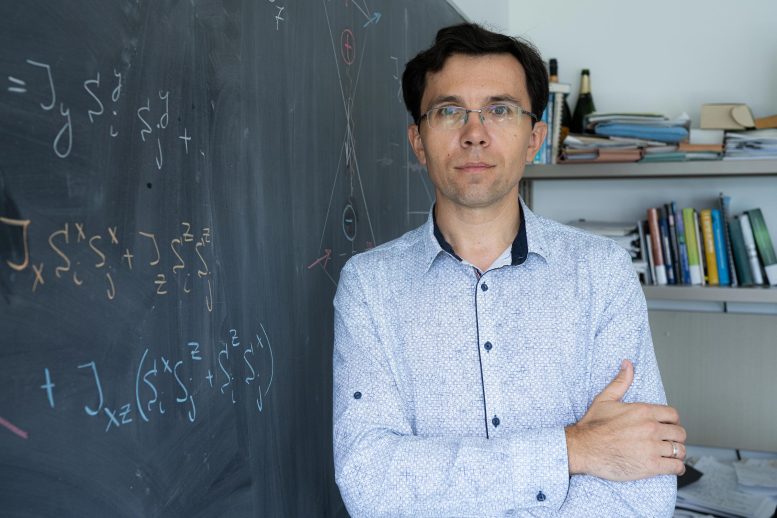
Spinons and Fractionalization
In quantum mechanics, electrons possess a property called spin, which behaves like a miniature bar magnet. When many electrons interact, their spins usually align or anti-align (aligning in an opposite direction). However, the arrangement of certain crystal structures such as pyrochlores can disrupt either arrangement. This phenomenon, called “magnetic frustration,” prevents spins from stabilizing into a conventional order, creating conditions where quantum mechanics can manifest in extraordinary ways, including the emergence of quantum spin liquids.
“Despite their name, quantum spin liquids exist in solid materials,” said Nevidomskyy, who has studied the quantum theory of frustrated magnets for years.
Nevidomskyy explained that the geometric frustration in a quantum spin liquid is so severe that the electrons instead form a quantum mechanical superposition that results in fluidlike correlations between electron spins as if the spins are immersed in a liquid.
Fractionalization and Quantum Electrodynamics
“What’s more, the elementary excitations are not an individual spin flipping its direction from up to down or vice versa,” Nevidomskyy said. “Instead, they are these bizarre, delocalized objects that carry half of one spin degree of freedom; we call them spinons. This phenomenon, when a single spin flip sort of splits into two halves, is called fractionalization.”
The concept of fractionalization and understanding how the resulting fractional particles interact with one another was key to the research performed by this experiment-theory collaboration. The spinons can be thought of as having a magnetic charge, and the interaction between two such particles is akin to electrically charged electrons repelling each other.
“At a quantum level, the electrons interact with one another by emitting and reabsorbing quanta of light known as photons. Similarly, in a quantum spin liquid, the interaction between spinons is described in terms of exchanging lightlike quanta,” said Nevidomskyy.
This analogy connects the study of quantum spin liquids with quantum electrodynamics (QED), the theory that describes how electrons interact through the exchange of photons and forms the foundation of the Standard Model of particle physics. Similarly, the theory of quantum pyrochlore magnets describes spinons as interacting via emergent “photons.” However, unlike QED in our universe, where light travels at a constant speed, the emergent “light” in these magnets is much slower — about 100 times slower than the speed of spinons. This stark difference leads to fascinating phenomena such as Cherenkov radiation and an increased likelihood of particle-antiparticle pair production. When combined with complementary research from a group of physicists at the University of Toronto, these findings offered unambiguous evidence for QED-like interactions in the experimental data.
“It is very exciting to see the difficult experiment and dedicated effort of theorists result in such a conclusion,” said Sibille.
Future Research and Applications
The study provides some of the clearest experimental evidence yet for quantum spin liquid states and their fractionalized excitations. It confirms that materials like cerium stannate can host these exotic phases of matter, which are not only fascinating for fundamental physics but could also have implications for quantum technologies like quantum computing. The results also suggest that we might be able to tune these materials to explore different quantum phenomena such as the existence of dual particles, opening doors to future research.
Dual particles, known as visons, are unlike spinons in that they carry an electric rather than magnetic charge. They resemble the theoretical magnetic monopoles first proposed nearly a century ago by quantum mechanics pioneer Paul Dirac, who predicted their quantization. Although magnetic monopoles have never been observed and are considered highly unlikely by high-energy theorists, the idea remains a captivating aspect of modern physics.
“After this discovery, it is all the more exciting to search for evidence of monopolelike particles in a toy universe formed out of electron spins in a piece of material,” said Nevidomskyy.
Reference: “Evidence for fractional matter coupled to an emergent gauge field in a quantum spin ice” by Victor Porée, Han Yan, Félix Desrochers, Sylvain Petit, Elsa Lhotel, Markus Appel, Jacques Ollivier, Yong Baek Kim, Andriy H. Nevidomskyy and Romain Sibille, 12 December 2024, Nature Physics.
DOI: 10.1038/s41567-024-02711-w
The research was supported by the Swiss National Science Foundation (R.S. and V.P., Grant No. 200021_179150), the U.S. National Science Foundation Division of Materials Research under the award DMR-1917511 (H.Y. and A.H.N.) and the Natural Sciences and Engineering Research Council of Canada (F.D. and YB.K.).
Leave a Reply