
By Ariana Tantillo and Haley Wahl, MIT Lincoln Laboratory December 13, 2024
Collected at: https://scitechdaily.com/illuminating-the-abyss-photon-rings-and-the-future-of-black-hole-imaging/
TBIRD, a laser communications system developed by Lincoln Laboratory, transmits data at unprecedented speeds, enabling scientists to capture black holes’ elusive photon rings.
The Event Horizon Telescope network captured groundbreaking images of a black hole in 2019 and 2021. Plans to enhance these observations include placing telescopes in space and employing advanced laser communication technology from MIT’s Lincoln Laboratory. This will enable detailed tests of gravitational theories through high-resolution imaging of black holes.
First Image of a Black Hole
In April 2019, astronomers from around the world made history by capturing the first-ever image of a black hole — a gravitational giant so powerful that not even light can escape its pull. This groundbreaking image revealed the glowing gas surrounding the supermassive black hole at the center of the galaxy Messier 87 (M87). Two years later, in March 2021, the same team released another remarkable image showing polarized light near the black hole, offering the first glimpse of its magnetic field.
The instrument behind these stunning images is the Event Horizon Telescope (EHT), a global network of radio telescopes that work together to form a virtual Earth-sized observatory. By combining data from telescopes around the globe, the EHT can create incredibly detailed images of distant black holes. Now, scientists are planning to extend the EHT into space to achieve even sharper views of M87’s black hole. However, transmitting the enormous amounts of data collected by space-based telescopes back to Earth is a major technical challenge. To solve this, researchers at MIT Lincoln Laboratory have developed a cutting-edge laser communications (lasercom) system capable of transmitting data at the extremely high speeds required for this ambitious mission.
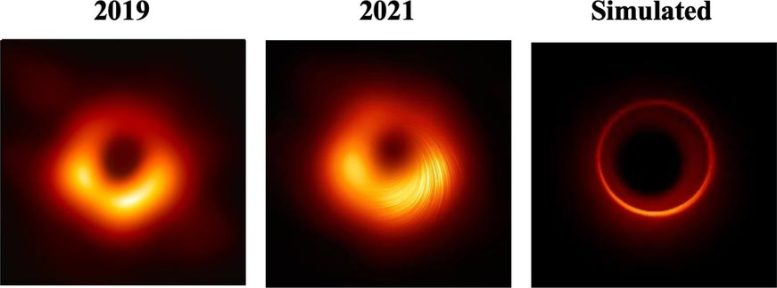
Credit: Event Horizon Telescope; Science Advances, vol. 6, no. 12
Expanding Imaging Techniques into Space
The EHT created the two existing images of M87’s black hole via interferometry — specifically, very long-baseline interferometry. Interferometry works by collecting light in the form of radio waves simultaneously with multiple telescopes in separate places on the globe and then comparing the phase difference of the radio waves at the various locations in order to pinpoint the direction of the source. By taking measurements with different combinations of the telescopes around the planet, the EHT collaboration — which included staff members at the Harvard-Smithsonian Center for Astrophysics (CfA) and MIT Haystack Observatory — essentially created an Earth-sized telescope in order to image the incredibly faint black hole 55 million light-years away from Earth.
With interferometry, the bigger the telescope, the better the resolution of the image. Therefore, in order to focus in on even finer characteristics of these black holes, a bigger instrument is needed. Details that astronomers hope to resolve include the turbulence of the gas falling into a black hole (which drives the accumulation of matter onto the black hole through a process called accretion) and a black hole’s shadow (which could be used to help pin down where the jet coming from M87 is drawing its energy from). The ultimate goal is to observe a photon ring (the place where light orbits closest before escaping) around the black hole. Capturing an image of the photon ring would enable scientists to put Albert Einstein’s general theory of relativity to the test.
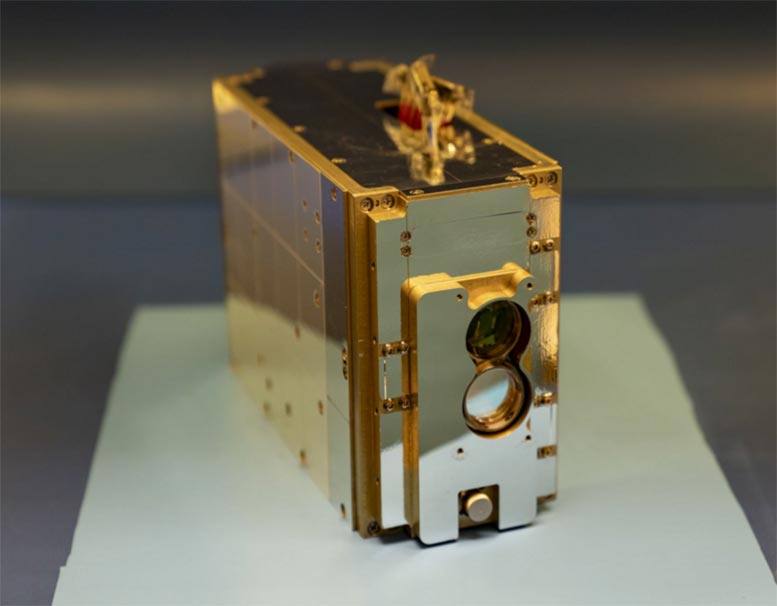
Advantages of Space-Based Observatories
With Earth-based telescopes, the farthest that two telescopes could be from one another is on opposite sides of the Earth, or about 13,000 kilometers apart. In addition to this maximum baseline distance, Earth-based instruments are limited by the atmosphere, which makes observing shorter wavelengths difficult. Earth’s atmospheric limitations can be overcome by extending the EHT’s baselines and putting at least one of the telescopes in space, which is exactly what the proposed CfA-led Black Hole Explorer (BHEX) mission aims to do.
One of the most significant challenges that comes with this space-based concept is transfer of information. The dataset to produce the first EHT image was so massive (totaling 4 petabytes) that the data had to be put on disks and shipped to a facility for processing. Gathering information from a telescope in orbit would be even more difficult; the team would need a system that can downlink data from the space telescope to Earth at approximately 100 gigabits per second (Gbps) in order to image the desired aspects of the black hole.
Breakthroughs in Data Transfer Technologies
Here is where Lincoln Laboratory comes in. In May 2023, the laboratory’s TeraByte InfraRed Delivery (TBIRD) lasercom payload achieved the fastest data transfer from space, transmitting at a rate of 200 Gbps — which is 1,000 times faster than typical satellite communication systems — from low Earth orbit (LEO).
“We developed a novel technology for high-volume data transport from space to ground,” says Jade Wang, assistant leader of the laboratory’s Optical and Quantum Communications Group. “In the process of developing that technology, we looked for collaborations and other potential follow-on missions that could leverage this unprecedented data capability. The BHEX is one such mission. These high data rates will enable scientists to image the photon ring structure of a black hole for the first time.”
A lasercom team led by Wang, in partnership with the CfA, is developing the long-distance, high-rate downlink needed for the BHEX mission in middle Earth orbit (MEO).
Future Prospects and Technology Development
“Laser communications is completely upending our expectations for what astrophysical discoveries are possible from space,” says CfA astrophysicist Michael Johnson, principal investigator for the BHEX mission. “In the next decade, this incredible new technology will bring us to the edge of a black hole, creating a window into the region where our current understanding of physics breaks down.”
Though TBIRD is incredibly powerful, the technology needs some modifications to support the higher orbit that BHEX requires for its science mission. The small TBIRD payload (CubeSat) will be upgraded to a larger aperture size and higher transmit power. In addition, the TBIRD automatic request protocol — the error-control mechanism for ensuring data make it to Earth without loss due to atmospheric effects — will be adjusted to account for the longer round-trip times that come with a mission in MEO. Finally, the TBIRD LEO “buffer and burst” architecture for data delivery will shift to a streaming approach.
“With TBIRD and other lasercom missions, we have demonstrated that the lasercom technology for such an impactful science mission is available today,” Wang says. “Having the opportunity to contribute to an area of really interesting scientific discovery is an exciting prospect.”
References:
“High Data Rate Laser Communications for the Black Hole Explorer” by Jade Wang, Bryan Bilyeu, Don Boroson, Dave Caplan, Kat Riesing, Bryan Robinson, Curt Schieler, Michael D. Johnson, Lindy Blackburn, Kari Haworth, Janice Houston, Sara Issaoun, Daniel Palumbo, Elliot Richards, Ranjani Srinivasan, Jonathan Weintroub and Dan Marrone, 13 June 2024, Astrophysics > Instrumentation and Methods for Astrophysics.
arXiv:2406.09572
“The Black Hole Explorer: Motivation and Vision” by Michael D. Johnson, Kazunori Akiyama, Rebecca Baturin, Bryan Bilyeu, Lindy Blackburn, Don Boroson, Alejandro Cardenas-Avendano, Andrew Chael, Chi-kwan Chan, Dominic Chang, Peter Cheimets, Cathy Chou, Sheperd S. Doeleman, Joseph Farah, Peter Galison, Ronald Gamble, Charles F. Gammie, Zachary Gelles, Jose L. Gomez, Samuel E. Gralla, Paul Grimes, Leonid I. Gurvits, Shahar Hadar, Kari Haworth, Kazuhiro Hada, Michael H. Hecht, Mareki Honma, Janice Houston, Ben Hudson, Sara Issaoun, He Jia, Svetlana Jorstad, Jens Kauffmann, Yuri Y. Kovalev, Peter Kurczynski, Robert Lafon, Alexandru Lupsasca, Robert Lehmensiek, Chung-Pei Ma, Daniel P. Marrone, Alan P. Marscher, Gary J. Melnick, Ramesh Narayan, Kotaro Niinuma, Scott C. Noble, Eric J. Palmer, Daniel C. M. Palumbo, Lenny Paritsky, Eliad Peretz, Dominic Pesce, Alexander Plavin, Eliot Quataert, Hannah Rana, Angelo Ricarte, Freek Roelofs, Katia Shtyrkova, Laura C. Sinclair, Jeffrey Small, Sridharan Tirupati Kumara, Ranjani Srinivasan, Andrew Strominger, Paul Tiede, Edward Tong, Jade Wang, Jonathan Weintroub, Maciek Wielgus, George Wong and Xinyue Alice Zhang, 13 June 2024, Astrophysics > Instrumentation and Methods for Astrophysics.
arXiv:2406.12917
The BHEX mission concept has been in development since 2019. Technical and concept studies for BHEX have been supported by the Smithsonian Astrophysical Observatory, the Internal Research and Development program at NASA Goddard Space Flight Center, the University of Arizona, and the ULVAC-Hayashi Seed Fund from the MIT-Japan Program at MIT International Science and Technology Initiatives. BHEX studies of lasercom have been supported by Fred Ehrsam and the Gordon and Betty Moore Foundation.
Leave a Reply