08 Dec 2021 by Michael Allen
Devices that exploit the extreme sensitivity of quantum states are making their way out of the lab and into everything from construction and healthcare to seismology. Michael Allen learns more about the technology that goes into building a quantum gravity sensor, and its multitude of uses in research and industry

Much of quantum technology is linked to computing. It is easy to imagine how a better, more powerful computer, capable of solving complex problems, could be useful. But what is a computer, after all, if not a data-processing machine. Computers, quantum or otherwise, transform data into information, which is then used to steer scientific, medical, industrial processes. Their output is only as good as the data put in. And those data are collected by sensors.
New kinds of sensors and novel ways of obtaining information have, in fact, triggered many major technological and economic disruptions. “If we look back in history, Nobel prizes are aligned with sensors, such as Wilhelm Röntgen’s 1901 prize for X-rays,” says Kai Bongs, a quantum physicist at the University of Birmingham, UK. “No-one knew what these were, but nowadays every hospital, airport scanner, as well as a number of industrial quality-control machines have X-ray machines in them. They allow us to see something, to get data inside bodies that we hadn’t been able to get before.”
Indeed, the discovery of X-rays had a significant impact on medicine, security and industrial processes. In a similar vein, sensors and other devices that exploit quantum principles – especially the sensitivity of a quantum state as compared to its environment – may well be the first stage of a commercial quantum revolution.
Bongs is principal investigator of the UK Quantum Technology Hub Sensors and Timing. This hub, which is part of the National Quantum Technologies Programme, has more than 110 projects valued at around £120m. Its aim is to drive the innovation and commercialization of quantum clocks and sensors such as magnetic sensors for healthcare and gravity sensors. These technologies have applications across a diverse range of sectors, including climate, communications, energy, transport, healthcare and urban development.
“When we talk about quantum sensors we mean sensors that use some enhanced quantum effects, like superposition or possibly entanglement,” says Bongs. Superposition is the idea that quantum particles can be in two states at once or travelling along two trajectories at once. “The difference between those two trajectories can create essentially a quantum interference at the end when we bring them back together and that allows us to read out what ever caused the difference with very high precision,” he explains.
This quantum effect is being exploited by Bongs and his colleagues to create a quantum gravity meter, based on atom interferometry. In the device, atoms travel along two different trajectories at two different heights. This means that they are travelling through two slightly different gravitational fields and when they come back together the interference pattern can be used to reveal the gravity gradient.
Gauging gravity
Most gravity meters are based on a mass suspended on a spring, with changes in the position of the mass indicating change in the gravitational forces acting on it. Springs, however, stretch and bounce around if the ground vibrates. This means that these devices have to be recalibrated over time. They also have to be left in place for quite a long time to take a reading, to average out the background noise created by vibrations due to everything from passing trucks and trains to low-level seismic activity and more.
Spring-based gravity meters are already very sensitive, but the advantage of a quantum gravity meter is that when the ground vibrates for any reason, the whole unit moves as one, as there is no spring to bounce around. The container, the clouds of atoms, and the laser that measures how they fall, all shift together. “You overcome not necessarily the sensitivity barrier,” Bongs says, “but you overcome the barrier of ground vibrations, so that you can measure much faster due to suppression of noise.” You can then start to push the sensitivity, he adds.
In civil engineering, gravity sensors can be used to detect anything under the ground that creates a mass difference. They could help find buried infrastructure such as pipes, tunnels and old mine shafts. While there are other techniques for doing this, such as ground penetrating radar, most of them are active techniques. You have to put a signal into the ground, which places limits on how deep they can go. “The real advantage of gravity is that it is passive, so the ground doesn’t attenuate the signal because we are not putting a signal in, we are just passively measuring it on the surface,” explains Daniel Boddice, a civil engineer at Birmingham. As long as the object produces a big enough signal on the surface, it can be detected. In theory there is no fundamental limit on depth.
Bad vibrations
Despite their potential, Boddice says that gravity sensors are not used very often, even in geophysics. This is because they are currently too expensive due to the amount of time you have to leave them in position to gather enough information so that you can cancel out the vibrational noise. He adds that there is always vibrational noise, because the Earth is always shaking. Boddice’s colleague Nicole Metje, also a civil engineer at Birmingham, says that it is not just seismic vibrations that create the noisy signal. “When you are on a site even things like traffic, people moving, people drilling, that all causes vibration.” Boddice adds. “The real opportunity with a quantum sensor is going to be that we can use it in more places because we can take measurements quicker and more viably, and more accurately.”
Metje and Boddice have recently been using quantum gravity sensors to detect culverts – a pipe or structure that channels water – on railway tracks. They provide drainage but if they become blocked, the track bed becomes saturated with water and you get what are known as “wet beds”. This impacts the track’s stability and can create structural issues such as dips, which affect the speed at which trains can safely travel, causing delays.
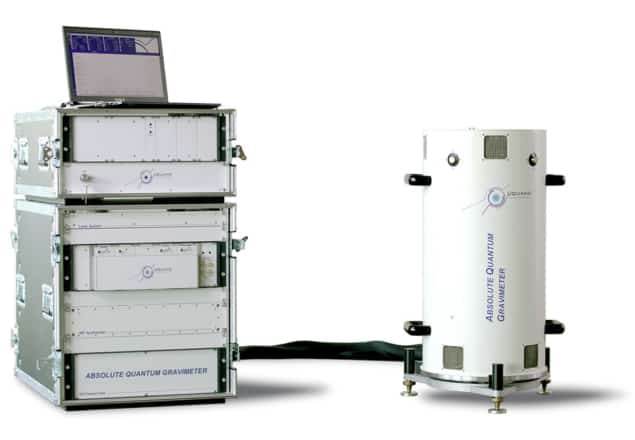
These culverts can be buried deep under the track, which can make finding them and assessing their condition challenging. Ground penetrating radar often cannot infiltrate far enough. Measurements also have to be taken quickly as engineers usually only have a few hours on the track at night. Metje has shown that gravity sensors work better than any other technique for finding railway culverts.
Existing spring-based gravity sensors are, however, a last resort, Metje points out, because they are slow. But quantum gravity sensors could change this. Not only could they take measurements much faster due to the lack of noise from vibrations, they also don’t need to be stationary. Such quantum sensors could be placed on trains scanning the tracks as they go – indeed, the Birmingham team has been running trails on sections of train track in the UK. “We’ve done some investigations where we have looked at silted culverts, full culverts and empty culverts – so full with water, full with silt and empty,” Metje says. “You can’t detect [the differences] if you just do one measurement, but the idea is that we could have sensors on trains and if you get time lapse measurements you can then detect differences.”
Mitigating risk
George Tuckwell at the RSK Group – a UK-based environmental and engineering consultancy – has also been exploring how these quantum gravity sensors could be used for civil engineering applications. RSK helps its clients “de-risk” construction projects by accessing ground conditions at an early stage. They map the ground to identify variations in bedrock and groundwater, and other natural and artificial variations in the ground such as landfill and mine workings. This protects projects against unforeseen problems that can cost money and cause delays.
Tuckwell and his colleagues already perform gravity surveys, but these can be slow, time consuming, and therefore expensive. They also almost always require follow-up work, such as digging a hole or drilling a borehole, to identify the mass anomaly. Tuckwell hopes that quantum sensors could change this, as they should be more accurate, much faster and able to measure things that current devices cannot.
Together with the University of Birmingham and other industrial partners, Tuckwell has been working on combining quantum gravity sensors with artificial intelligence, past data and machine learning to create a system that can provide a model of the most probable ground conditions. The ultimate goal is to create a set-up that can scan a patch of land and provide an accurate map of the subsurface, with all the required information and no need for further investigations.
One of the advantages gravity has as a sensor is that it is impossible to shield a subsurface feature from its gravitational affect, so there is no way of hiding it. If there is something there that gravity could measure then gravity will measure itGeorge Tuckwell, RSK Group
“You can imagine an intermediate step where you would scan the ground, and the gravity sensor would say these are the three things that [the mass anomaly] is most likely to be and this is what you should do to confirm or otherwise to pin it down to one possibility – and lead you through what follow-up data to take,” Tuckwell says. “We have already developed an algorithm that as you are doing the survey it can suggest to you where you should measure the next point, to have the biggest impact on the certainty you’ll get of the model of the subsurface, and you can do that as you are going along.”
Although civil engineering is going to see the first commercial applications of gravity sensors, Tuckwell adds that there is also a lot of military interest. “For a defence and security application it might be a clandestine tunnel or some subsurface facility,” he explains. “And one of the advantages gravity has as a sensor is that it is impossible to shield a subsurface feature from its gravitational affect, so there is no way of hiding it. If there is something there that gravity could measure then gravity will measure it.”
From ships to volcanoes
Quantum gravity meters could also be used to create secure navigation systems. In recent years there has been increasing concern about GPS spoofing, particularly in marine navigation. This is when a ship’s navigation system is sent a false signal, so that it thinks it is in a different position to where it actually is. In the hands of rogue states or pirates this could potentially be used to hijack or wreck boats, or steer them into hostile territorial waters – a 21st-century version of the lanterns used by Cornish ship-wreckers.
If we could create accurate gravity maps, ships could have an onboard quantum gravity meter, and could then trace the gravity values and match them to the map, giving them their position. In theory, this gravity meter could be in an enclosed box entirely isolated from the outside world, making it unhackable. Even if somebody jammed the ship’s communications, satellite and radar navigation systems – all its links to the outside world – it would still be able to navigate. “The only way to interfere with [the gravity sensor] is to essentially change the gravitational signal, but that means moving masses on the order of mountains,” Bongs explains.
Indeed, gravity meters can be used “to detect the invisible”, according to Bruno Desruelle – chief executive of French spin-off company μQUANS (Muquans), which specializes in high-precision quantum measurements. While the firm has a number of laser and cold-atom engineering products and atomic clocks, its quantum gravimeters are already being used out in the field – for example, it is working with the French Ministry of Defence to supply shipborne quantum gravimeters. But more excitingly, Muquans has developed its Absolute Quantum Gravimeter, which is currently situated near the top of Mount Etna in Italy. The system has now been collecting data on the slopes of the Sicilian volcano for about a year.
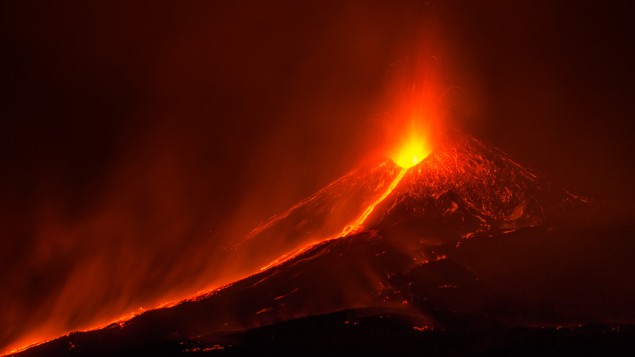
Measuring changes in gravity around a volcano is essential as it gives clues about changes in density of the underlying materials such as rock, gas and magma. An increase in gravity might suggest an influx of a denser material such as magma, while a drop in density (and so gravity) would point to a sinkhole. “The idea behind this instrument is really to exploit the gravity measurement at the surface of the volcano in order to obtain some information about the underground geophysical processes and get a better understanding of what is happening inside the volcano,” Desruelle explains. The main thing the device is measuring is changes in masses of magma inside the volcano, with the long-term goal of gathering enough data to predict volcanic eruptions. He adds that this is likely the first time that geophysicists have deployed a gravimeter so close to the top of a volcano, and that the researchers have already acquired some new information about the behaviour of Mount Etna, which will be published soon.
According to Desruelle, the way a quantum gravity meter works is both complicated and simple. Simple because it is basically a Newton experiment, where the test mass is dropped in a vacuum chamber and its vertical acceleration is measured, to give the value of gravitational acceleration, g, at any point on the Earth’s surface. Where is gets complicated is that instead of using an apple, this device uses a cloud of laser-cooled rubidium atoms. Every half a second, it captures a cloud of atoms, cools them to a temperature of a few kelvin and then lets them fall, using lasers to measure their acceleration (Sci. Rep. 8 12300).
The quantum nature of the device comes from its reliance on wave–particle duality and using quantum interference to reveal differences in the gravitational field. The company did have to struggle with some challenges when it came to the mass, power consumption and size of the device. These factors were especially important for being able to successfully deploy the gravimeter in terrain such as that of Etna, making it robust enough to survive a year or more of changing temperatures.
As with many civil-engineering applications, the lack of noise from vibrations is a big advantage on a volcano. “As you may guess, the volcano is not a very friendly environment,” Desruelle says. “You have a very strong level of vibrations all the time and it is very challenging to acquire a very high resolution on the gravity measurement.” Another advantage is the lack of any springs in the quantum system, meaning that measurements can be taken for a very long time, with no need to recalibrate the system. Being able to collect data without any interruptions for months or years is very important for understanding complex geophysical systems – and is simply not possible with a mechanical gravity meter.
Beyond computing
Desruelle believes that while advances in building quantum computers are very interesting, “we are still talking about research activities and long-term perspectives”. Quantum gravity meters, however, are already operational and being used in the field. “I really believe that with quantum gravity meters, we have reached a very different level of technological maturity,” he says, pointing to concrete examples of their applications. Quantum gravity sensors can be used for any activity where “you really want to have an idea of the underground mass distribution”. This applies to hydrology and seismology, as well as civil engineering projects to detect voids, sink holes, tunnels and cavities. “Many people are interested in this instrument for geodesy,” he adds, “so they want to get a very good understanding of the geosphere and the gravity maps, so many institutes are assigned to provide gravity maps in many areas.”
A somewhat less practical, but equally interesting from a research point of view, application is that gravity meters can be used as dark-matter detectors. For civil engineering and geophysical applications, the two different paths the atom follows are just a few millimetres apart, but increasing the size of the device significantly increases its sensitivity, allowing it to be used to detect certain candidates for this mysterious invisible matter, which makes up almost 85% of matter in the universe.
As of January, UK Research and Innovation had already funded seven projects, with a £31m investment, that hope to show how quantum technologies could solve some of the greatest mysteries in fundamental physics. Three of the projects are looking at developing quantum-enhanced interferometry and sensors in the hunt for dark matter – to detect ultra-light candidates such as axions or to test our theories about the quantization of space–time. Often, a paradigm-shifting discovery in science comes on the back of melding together new technologies and established theories. We might still be at the very dawn of quantum sensors, but how remarkable it is that they can simultaneously help us build a better road and delve into the deepest mysteries of the universe.