
19 Dec 2024 Hamish Johnston
Collected at: https://physicsworld.com/a/two-advances-in-quantum-error-correction-share-the-physics-world-2024-breakthrough-of-the-year/
The Physics World 2024 Breakthrough of the Year goes to Mikhail Lukin, Dolev Bluvstein and colleagues at Harvard University, the Massachusetts Institute of Technology and QuEra Computing, and independently to Hartmut Neven and colleagues at Google Quantum AI and their collaborators, for demonstrating quantum error correction on an atomic processor with 48 logical qubits, and for implementing quantum error correction below the surface code threshold in a superconducting chip, respectively.
Errors caused by interactions with the environment – noise – are the Achilles heel of every quantum computer, and correcting them has been called a “defining challenge” for the technology. These two teams, working with very different quantum systems, took significant steps towards overcoming this challenge. In doing so, they made it far more likely that quantum computers will become practical problem-solving machines, not just noisy, intermediate-scale tools for scientific research.
Quantum error correction works by distributing one quantum bit of information – called a logical qubit – across several different physical qubits such as superconducting circuits or trapped atoms. While each physical qubit is noisy, they work together to preserve the quantum state of the logical qubit – at least for long enough to do a computation.
Formidable task
Error correction should become more effective as the number of physical qubits in a logical qubit increases. However, integrating large numbers of physical qubits to create a processor with multiple logical qubits is a formidable task. Furthermore, adding more physical qubits to a logical qubit also adds more noise – and it is not clear whether making logical qubits bigger would make them significantly better. This year’s winners of our Breakthrough of the Year have made significant progress in addressing these issues.
The team led by Lukin and Bluvstein created a quantum processor with 48 logical qubits that can execute algorithms while correcting errors in real time. At the heart of their processor are arrays of neutral atoms. These are grids of ultracold rubidium atoms trapped by optical tweezers. These atoms can be put into highly excited Rydberg states, which enables the atoms to act as physical qubits that can exchange quantum information.
What is more, the atoms can be moved about within an array to entangle them with other atoms. According to Bluvstein, moving groups of atoms around the processor was critical for their success at addressing a major challenge in using logical qubits: how to get logical qubits to interact with each other to perform quantum operations. He describes the system as a “living organism that changes during a computation”.
Their processor used about 300 physical qubits to create up to 48 logical qubits, which were used to perform logical operations. In contrast, similar attempts using superconducting or trapped-ion qubits have only managed to perform logical operations using 1–3 logical qubits.
Willow quantum processor
Meanwhile, the team led by Hartmut Neven made a significant advance in how physical qubits can be combined to create a logical qubit. Using Google’s new Willow quantum processor – which offers up to 105 superconducting physical qubits – they showed that the noise in their logical qubit remained below a maximum threshold as they increased the number of qubits. This means that the logical error rate is suppressed exponentially as the number of physical qubits per logical qubit is increased.
Neven told Physics World that the Google system is “the most convincing prototype of a logical qubit built today”. He said that that Google is on track to develop a quantum processor with 100 or even 1000 logical qubits by 2030. He says that a 1000 logical qubit device could do useful calculations for the development of new drugs or new materials for batteries.
Bluvstein, Lukin and colleagues are already exploring how their processor could be used to study an effect called quantum scrambling. This could shed light on properties of black holes and even provide important clues about the nature of quantum gravity.
You can listen to Neven talk about his team’s research in this podcast. Bluvstein and Lukin talk about their group’s work in this podcast.
The Breakthrough of the Year was chosen by the Physics World editorial team. We looked back at all the scientific discoveries we have reported on since 1 January and picked the most important. In addition to being reported in Physics World in 2024, the breakthrough must meet the following criteria:
- Significant advance in knowledge or understanding
- Importance of work for scientific progress and/or development of real-world applications
- Of general interest to Physics World readers
Before we picked our winners, we released the Physics World Top 10 Breakthroughs for 2024, which served as our shortlist. The other nine breakthroughs are listed below in no particular order.
Light-absorbing dye turns skin of live mouse transparent
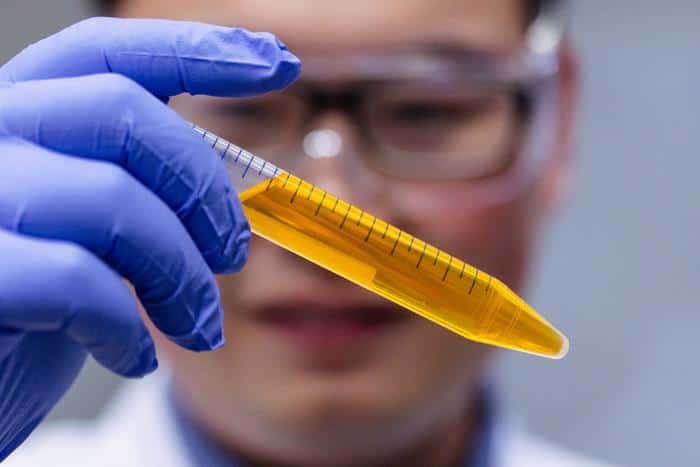
To a team of researchers at Stanford University in the US for developing a method to make the skin of live mice temporarily transparent. One of the challenges of imaging biological tissue using optical techniques is that tissue scatters light, which makes it opaque. The team, led by Zihao Ou (now at The University of Texas at Dallas), Mark Brongersma and Guosong Hong, found that the common yellow food dye tartrazine strongly absorbs near-ultraviolet and blue light and can help make biological tissue transparent. Applying the dye onto the abdomen, scalp and hindlimbs of live mice enabled the researchers to see internal organs, such as the liver, small intestine and bladder, through the skin without requiring any surgery. They could also visualize blood flow in the rodents’ brains and the fine structure of muscle sarcomere fibres in their hind limbs. The effect can be reversed by simply rinsing off the dye. This “optical clearing” technique has so far only been conducted on animals. But if extended to humans, it could help make some types of invasive biopsies a thing of the past.
Laser cooling positronium
To the AEgIS collaboration at CERN, and Kosuke Yoshioka and colleagues at the University of Tokyo, for independently demonstrating laser cooling of positronium. Positronium, an atom-like bound state of an electron and a positron, is created in the lab to allow physicists to study antimatter. Currently, it is created in “warm” clouds in which the atoms have a large distribution of velocities, making precision spectroscopy difficult. Cooling positronium to low temperatures could open up novel ways to study the properties of antimatter. It also enables researchers to produce one to two orders of magnitude more antihydrogen – an antiatom comprising a positron and an antiproton that’s of great interest to physicists. The research also paves the way to use positronium to test current aspects of the Standard Model of particle physics, such as quantum electrodynamics, which predicts specific spectral lines, and to probe the effects of gravity on antimatter.
Modelling lung cells to personalize radiotherapy
To Roman Bauer at the University of Surrey, UK, Marco Durante from the GSI Helmholtz Centre for Heavy Ion Research, Germany, and Nicolò Cogno from GSI and Massachusetts General Hospital/Harvard Medical School, US, for creating a computational model that could improve radiotherapy outcomes for patients with lung cancer. Radiotherapy is an effective treatment for lung cancer but can harm healthy tissue. To minimize radiation damage and help personalize treatment, the team combined a model of lung tissue with a Monte Carlo simulator to simulate irradiation of alveoli (the tiny air sacs within the lungs) at microscopic and nanoscopic scales. Based on the radiation dose delivered to each cell and its distribution, the model predicts whether each cell will live or die, and determines the severity of radiation damage hours, days, months or even years after treatment. Importantly, the researchers found that their model delivered results that matched experimental observations from various labs and hospitals, suggesting that it could, in principle, be used within a clinical setting.
A semiconductor and a novel switch made from graphene

To Walter de Heer, Lei Ma and colleagues at Tianjin University and the Georgia Institute of Technology, and independently to Marcelo Lozada-Hidalgo of the University of Manchester and a multinational team of colleagues, for creating a functional semiconductor made from graphene, and for using graphene to make a switch that supports both memory and logic functions, respectively. The Manchester-led team’s achievement was to harness graphene’s ability to conduct both protons and electrons in a device that performs logic operations with a proton current while simultaneously encoding a bit of memory with an electron current. These functions are normally performed by separate circuit elements, which increases data transfer times and power consumption. Conversely, de Heer, Ma and colleagues engineered a form of graphene that does not conduct as easily. Their new “epigraphene” has a bandgap that, like silicon, could allow it to be made into a transistor, but with favourable properties that silicon lacks, such as high thermal conductivity.
Detecting the decay of individual nuclei
To David Moore, Jiaxiang Wang and colleagues at Yale University, US, for detecting the nuclear decay of individual helium nuclei by embedding radioactive lead-212 atoms in a micron-sized silica sphere and measuring the sphere’s recoil as nuclei escape from it. Their technique relies on the conservation of momentum, and it can gauge forces as small as 10-20 N and accelerations as tiny as 10-7 g, where g is the local acceleration due to the Earth’s gravitational pull. The researchers hope that a similar technique may one day be used to detect neutrinos, which are much less massive than helium nuclei but are likewise emitted as decay products in certain nuclear reactions.
Two distinct descriptions of nuclei unified for the first time
To Andrew Denniston at the Massachusetts Institute of Technology in the US, Tomáš Ježo at Germany’s University of Münster and an international team for being the first to unify two distinct descriptions of atomic nuclei. They have combined the particle physics perspective – where nuclei comprise quarks and gluons – with the traditional nuclear physics view that treats nuclei as collections of interacting nucleons (protons and neutrons). The team has provided fresh insights into short-range correlated nucleon pairs – which are fleeting interactions where two nucleons come exceptionally close and engage in strong interactions for mere femtoseconds. The model was tested and refined using experimental data from scattering experiments involving 19 different nuclei with very different masses (from helium-3 to lead-208). The work represents a major step forward in our understanding of nuclear structure and strong interactions.
New titanium:sapphire laser is tiny, low-cost and tuneable
To Jelena Vučković, Joshua Yang, Kasper Van Gasse, Daniil Lukin, and colleagues at Stanford University in the US for developing a compact, integrated titanium:sapphire laser that needs only a simple green LED as a pump source. They have reduced the cost and footprint of a titanium:sapphire laser by three orders of magnitude and the power consumption by two. Traditional titanium:sapphire lasers have to be pumped with high-powered lasers – and therefore cost in excess of $100,000. In contrast, the team was able to pump its device using a $37 green laser diode. The researchers also achieved two things that had not been possible before with a titanium:sapphire laser. They were able to adjust the wavelength of the laser light and they were able to create a titanium:sapphire laser amplifier. Their device represents a key step towards the democratization of a laser type that plays important roles in scientific research and industry.
Entangled photons conceal and enhance images
To two related teams for their clever use of entangled photons in imaging. Both groups include Chloé Vernière and Hugo Defienne of Sorbonne University in France, who as duo used quantum entanglement to encode an image into a beam of light. The impressive thing is that the image is only visible to an observer using a single-photon sensitive camera – otherwise the image is hidden from view. The technique could be used to create optical systems with reduced sensitivity to scattering. This could be useful for imaging biological tissues and long-range optical communications. In separate work, Vernière and Defienne teamed up with Patrick Cameron at the UK’s University of Glasgow and others to use entangled photons to enhance adaptive optical imaging. The team showed that the technique can be used to produce higher-resolution images than conventional bright-field microscopy. Looking to the future, this adaptive optics technique could play a major role in the development of quantum microscopes.
First samples returned from the Moon’s far side
To the China National Space Administration for the first-ever retrieval of material from the Moon’s far side, confirming China as one of the world’s leading space nations. Landing on the lunar far side – which always faces away from Earth – is difficult due to its distance and terrain of giant craters with few flat surfaces. At the same time, scientists are interested in the unexplored far side and why it looks so different from the near side. The Chang’e-6 mission was launched on 3 May consisting of four parts: an ascender, lander, returner and orbiter. The ascender and lander successfully touched down on 1 June in the Apollo basin, which lies in the north-eastern side of the South Pole-Aitken Basin. The lander used its robotic scoop and drill to obtain about 1.9 kg of materials within 48 h. The ascender then lifted off from the top of the lander and docked with the returner-orbiter before the returner headed back to Earth, landing in Inner Mongolia on 25 June. In November, scientists released the first results from the mission finding that fragments of basalt – a type of volcanic rock – date back to 2.8 billion years ago, indicating that the lunar far side was volcanically active at that time. Further scientific discoveries can be expected in the coming months and years ahead as scientists analyze more fragments.
Leave a Reply