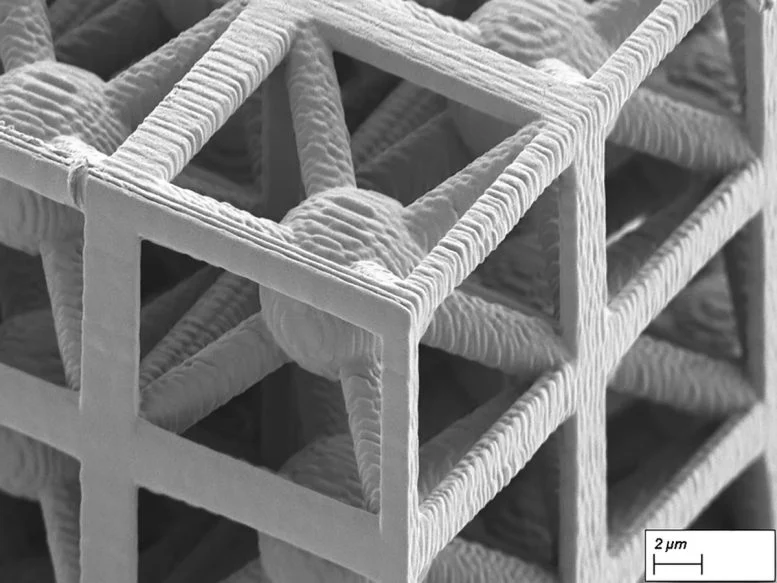
By Anne Wilson, Massachusetts Institute of Technology December 14, 2024
Collected at: https://scitechdaily.com/sonic-breakthrough-mit-unlocks-ultrasound-control-with-advanced-metamaterials/
Researchers at MIT have developed a design framework for controlling ultrasound wave propagation in microscale acoustic metamaterials, focusing on the precise positioning of microscale spheres within a lattice.
This approach enables tunable wave velocities and responses, and is applicable in fields like ultrasound imaging and mechanical computing.
Acoustic Metamaterials
Acoustic metamaterials are specially designed materials with carefully engineered structures that control how sound or elastic waves move through them. While researchers have explored these materials through computer models and theoretical studies, creating physical versions has so far been limited to large-scale structures and low-frequency applications.
“The multifunctionality of metamaterials — being simultaneously lightweight and strong while having tunable acoustic properties — make them great candidates for use in extreme-condition engineering applications,” explains Carlos Portela, the Robert N. Noyce Career Development Chair and assistant professor of mechanical engineering at MIT. “But challenges in miniaturizing and characterizing acoustic metamaterials at high frequencies have hindered progress towards realizing advanced materials that have ultrasonic-wave control capabilities.”
New Design Framework for Ultrasound Wave Control
Portela, along with Rachel Sun, Jet Lem, and Yun Kai from MIT’s Department of Mechanical Engineering, and Washington DeLima from the U.S. Department of Energy’s Kansas City National Security Campus, recently developed a new design framework for controlling ultrasound waves in microscopic acoustic metamaterials. Their findings, detailed in the paper “Tailored Ultrasound Propagation in Microscale Metamaterials via Inertia Design,” were published in the journal Science Advances.
“Our work proposes a design framework based on precisely positioning microscale spheres to tune how ultrasound waves travel through 3D microscale metamaterials,” says Portela. “Specifically, we investigate how placing microscopic spherical masses within a metamaterial lattice affects how fast ultrasound waves travel throughout, ultimately leading to wave guiding or focusing responses.”
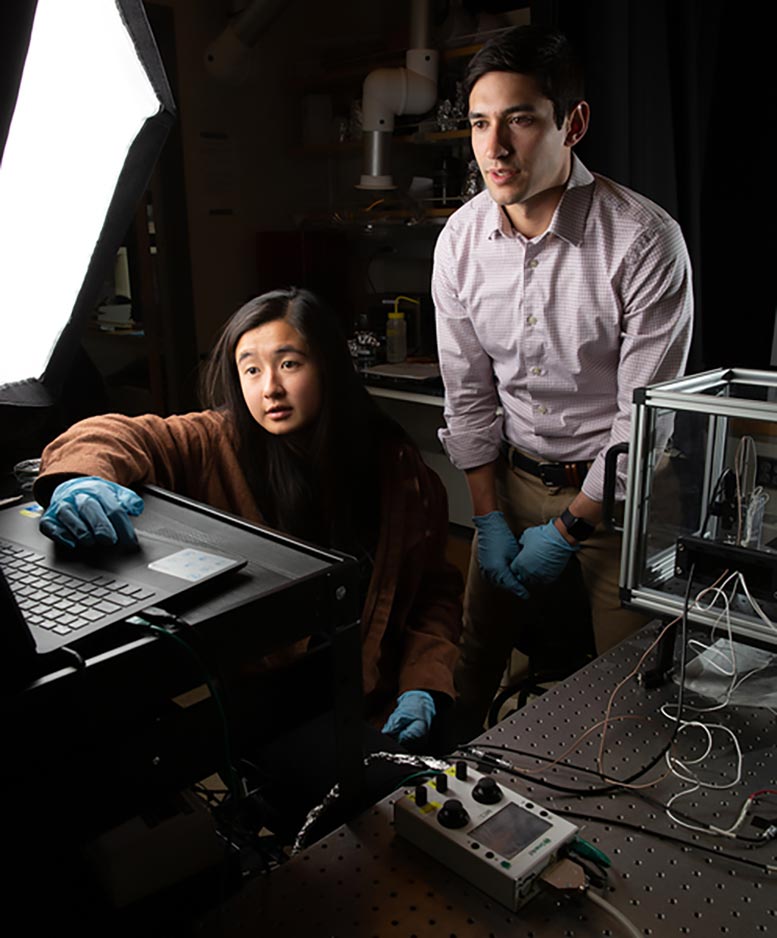
Advancements in Metamaterial Technology
Through non-destructive, high-throughput laser-ultrasonics characterization, the team experimentally demonstrates tunable elastic-wave velocities within microscale materials. They use the varied wave velocities to spatially and temporally tune wave propagation in microscale materials, also demonstrating an acoustic demultiplexer (a device that separates one acoustic signal into multiple output signals). The work paves the way for microscale devices and components that could be useful for ultrasound imaging or information transmission via ultrasound.
“Using simple geometrical changes, this design framework expands the tunable dynamic property space of metamaterials, enabling straightforward design and fabrication of microscale acoustic metamaterials and devices,” says Portela.
Broad Applications and Future Potential
The research also advances experimental capabilities, including fabrication and characterization, of microscale acoustic metamaterials toward application in medical ultrasound and mechanical computing applications, and underscores the underlying mechanics of ultrasound wave propagation in metamaterials, tuning dynamic properties via simple geometric changes and describing these changes as a function of changes in mass and stiffness. More importantly, the framework is amenable to other fabrication techniques beyond the microscale, requiring merely a single constituent material and one base 3D geometry to attain largely tunable properties.
“The beauty of this framework is that it fundamentally links physical material properties to geometric features. By placing spherical masses on a spring-like lattice scaffold, we could create direct analogies for how mass affects quasi-static stiffness and dynamic wave velocity,” says Sun, first author of the study. “I realized that we could obtain hundreds of different designs and corresponding material properties regardless of whether we vibrated or slowly compressed the materials.”
Reference: “Tailored ultrasound propagation in microscale metamaterials via inertia design” by Rachel Sun, Jet Lem, Yun Kai, Washington DeLima and Carlos M. Portela, 6 November 2024, Science Advances.
DOI: 10.1126/sciadv.adq6425
This work was carried out, in part, through the use of MIT.nano facilities.
Leave a Reply