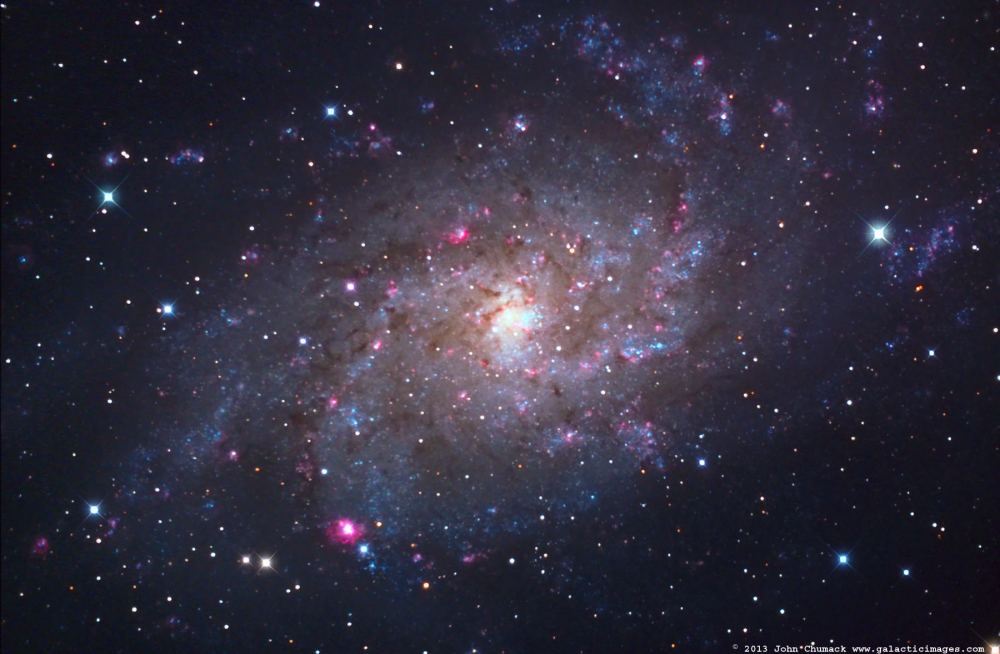
October 25, 2024 by Matt Williams
Collected at: https://www.universetoday.com/168913/webb-finds-dozens-of-supernovae-remnants-in-the-triangulum-galaxy/
Infrared astronomy has revealed so much about the Universe, ranging from protoplanetary disks and nebulae to brown dwarfs, aurorae, and volcanoes on together celestial bodies. Looking to the future, astronomers hope to conduct infrared studies of supernova remnants (SNRs), which will provide vital information about the physics of these explosions. While studies in the near-to-mid infrared (NIR-MIR) spectrum are expected to provide data on the atomic makeup of SNRs, mid-to-far IR (MIR-FIR) studies should provide a detailed look at heated dust grains they eject into the interstellar medium (ISM).
Unfortunately, these studies have been largely restricted to the Milky Way and the Magellanic Clouds due to the limits of previous IR observatories. However, these observational regimes are now accessible thanks to next-generation instruments like the James Webb Space Telescope (JWST). In a recent study, a team led by researchers from Ohio State University presented the first spatially resolved infrared images of supernova remnants (SNRs) in the Triangulum Galaxy (a.k.a. Messier 33). Their observations allowed them to acquire images of 43 SNRs, thanks to the unprecedented sensitivity and resolution of Webb’s IR instruments.
The team was led by Dr. Sumit K. Sarbadhicary, a former Postdoctoral Fellow with OSU’s Center for Cosmology & Astro-Particle Physics (CCAP) and current Assistant Research Scientist at Johns Hopkins University (JHU). He was joined by multiple astronomers and physicists from OSU, the Harvard & Smithsonian Center for Astrophysics, the Flatiron Institute’s Center for Computational Astrophysics, the University of Heidelberg’s Institute for Theoretical Astrophysics, the National Radio Astronomy Observatory (NRAO), and the Space Telescope Science Institute (STScI). The paper that describes their findings is being reviewed for publication in The Astrophysical Journal.
![The NASA/ESA/CSA James Webb Space Telescope has gazed at the Crab Nebula in the search for answers about the supernova remnant’s origins. Webb’s NIRCam (Near-Infrared Camera) and MIRI (Mid-Infrared Instrument) have revealed new details in infrared light. Similar to the Hubble optical wavelength image released in 2005, with Webb the remnant appears to consist of a crisp, cage-like structure of fluffy red-orange filaments of gas that trace doubly ionised sulphur (sulphur III). Within the remnant’s interior, yellow-white and green fluffy ridges form large-scale loop-like structures, which represent areas where dust particles reside. The area is composed of translucent, milky material. This material is emitting synchrotron radiation, which is emitted across the electromagnetic spectrum but becomes particularly vibrant thanks to Webb’s sensitivity and spatial resolution. It is generated by particles accelerated to extremely high speeds as they wind around magnetic field lines. The synchrotron radiation can be traced throughout the majority of the Crab Nebula’s interior. Locate the wisps that follow a ripple-like pattern in the middle. In the centre of this ring-like structure is a bright white dot: a rapidly rotating neutron star. Further out from the core, follow the thin white ribbons of the radiation. The curvy wisps are closely grouped together, following different directions that mimic the structure of the pulsar’s magnetic field. Note how certain gas filaments are bluer in colour. These areas contain singly ionised iron (iron II). [Image description: An oval nebula with a complex structure against a black background. On the oval's exterior lie curtains of glowing red and orange fluffy material. Interior to this outer shell lie large-scale loops of mottled filaments of yellow-white and green, studded with clumps and knots. Translucent thin ribbons of smoky white lie within the remnant’s interior, brightest toward its centre.]](https://www.universetoday.com/wp-content/uploads/2023/10/Crab-1-1024x892.jpeg)
As they explain in their study, SNRs in the Milky Way and Magellanic clouds are the best studied in the Universe because they are the closest. This has allowed astronomers to conduct detailed studies that revealed their structures at most wavelengths, including infrared. As Dr. Sarbadhicary told Universe Today via email, studies of these SNRs have taught astronomers a great deal. This includes dust production, the composition of supernova explosions, and the physics of astrophysical shock waves – particularly those that travel through dense gas clouds where new stars could be forming.
However, as Sarbadhicary explained, these studies have still been confined to our galaxy and its satellites, which has limited what astronomers can learn about these major astronomical events:
“[The] only thing is, we haven’t quite been able to step outside the Magellanic Clouds and explore SNRs in more distant galaxies in the infrared. We know that other Local Group galaxies such as Andromeda (M31), and Triangulum (M33) have several hundreds of SNRs, so there is a tremendous potential for building statistics. Additionally, infrared-emitting SNRs are a somewhat rare breed, found mostly in explosions that happened close to dense molecular gas that is either part of the interstellar medium, or material lost by the progenitor star before explosion. So having more objects would be really helpful.”
The first generation of SNR studies at infrared wavelengths were conducted with NASA’s Infrared Astronomical Satellite (IRAS) and the ESA’s Infrared Space Observatory (ISO). Despite their limited spatial resolution and the confusion of peering through the Galactic plane, these observatories managed to identify about 30% of SNRs in the Milky Way between 10 and 100 micrometers (?m), which corresponds to parts of the Medium and Far-Infrared (MIR, NIR) spectrum.

In recent decades, IR astronomy has benefitted immensely from missions like NASA’s Spitzer Space Telescope and the ESA’s Herschel Space Observatory. These observatories boast higher angular resolutions and can conduct surveys in broader parts of the IR spectrum – 3 to 160 ?m for Spitzer and 70 to 500 ?m for Herschel. Their observations led to wide-field Galactic surveys – the Galactic Legacy Infrared Midplane Survey Extraordinaire (GLIMPSE), the MIPS Galactic Plane Survey (MIPSGAL), and the Herschel infrared Galactic Plane Survey (Hi-GAL) – and the first high-quality extragalactic IR surveys of SNRs.
“Unfortunately, the angular resolution of the Spitzer telescope (JWST’s predecessor) was just not good enough to recover the same spatial detail in more distant galaxies,” added Sarbadhicary. “While you might see a faint blip with Spitzer, it would be hard to tell (at these distances) if it’s from the SNR or some blend of stars and diffuse emission.” Fortunately, the situation has improved even more with the deployment of the James Webb Space Telescope (JWST). According to Sarbadhicary, Webb’s increased resolution and advanced IR instruments are providing deeper and sharper views of SNRs in the near- and mid-infrared wavelengths:
“We had already seen JWST’s potential for revolutionizing studies of SNRs from crisp new images of known SNRs such as Cassiopeia A in our Galaxy and 1987A in the Large Magellanic Cloud, published in recent papers. The images revealed an unprecedented amount of detail about the explosion debris, material lost by the star prior to the explosion, and much more.
“This superior combination of sensitivity and angular resolution also now enables JWST to recover images of SNRs in galaxies nearly 20 times farther than the Magellanic Clouds (e.g., M33 in our paper), with the same level of detail found by Spitzer in SNRs in the Magellanic Clouds. What is particularly helpful because of JWST’s high angular resolution is that we are less likely to confuse SNRs with overlapping structures such as HII regions (gas photoionized by massive stars).”
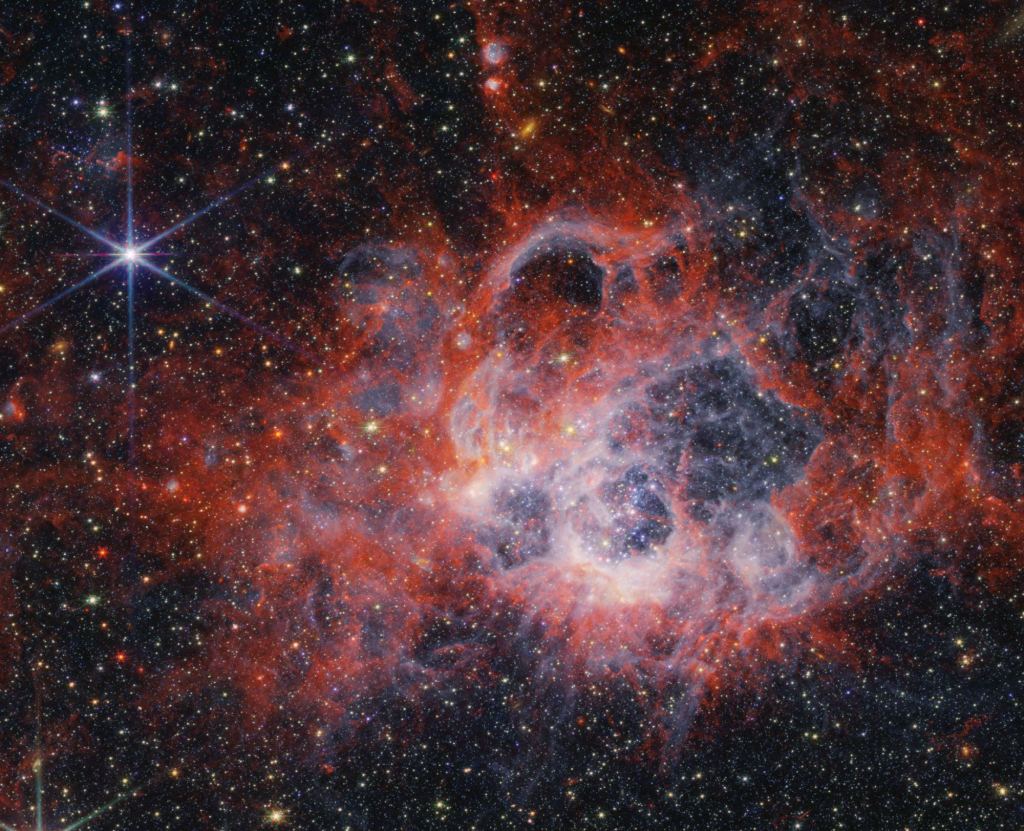
For their study, Sarbadhicary and his team leveraged archival JWST observations of the Trangulum Galaxy (M33) in four JWST fields. Two of these covered central and southern regions of M33 with separate observations using Webb’s Near-Infrared Camera (NIRCam) and its Mid-Infrared Imager (MIRI). The third involved MIRI observations of a long radial strip measuring about 5 kiloparsecs (~16,300 light-years), one covering the giant emission nebula in M33 (NGC 604) with multiple NIRCam and MIRI observations. They then overlapped these observations with previously identified SNRs from multi-wavelength surveys.
They also considered the volumes of multi-wavelength data previous missions have obtained of this galaxy. This includes images of stars acquired by the venerable Hubble and cold neutral gas observations conducted by the Atacama Large Millimeter-submillimeter Array (ALMA) and the Very Large Array (VLA). As Sarbadhicary indicated, the results revealed some very interesting things about SNRs in the Triangulum Galaxy. However, since their survey covered only 20% of the SNRs in M33, he also noted that these results are just the tip of the iceberg:
“The most surprising finding was the presence of molecular hydrogen emission in two out of the three SNRs where we had F470N observations (a narrowband filter centered on the 4.7-micron rotational line of the hydrogen molecule). Molecular hydrogen is by far the most abundant molecule in interstellar gas, but because of the symmetry of the molecule, it cannot produce visible radiation at the typical cold temperatures of interstellar gas. Only when heated by shocks or ultraviolet emission does H2 emit radiation (such as at 4.7 microns), so it is a very useful tracer of shocks hitting dense molecular gas, where star formation occurs.”
While astronomers have seen this emission in several SNRs within the Milky Way, this was the first time such observations have been made of an extragalactic source. “The JWST data also revealed that between 14-43% of the SNRs show visible infrared emission,” added Sarbadhicary. “The brightest infrared SNRs in our sample are also some of the smallest in M33 and the brightest at other wavelengths, especially X-ray, radio, and optical. This means that the shocks in these SNRs are still traveling relatively fast and hitting high-density material in the environment, leading to a substantial amount of the shock energy being radiated into infrared lines and dust that are illuminating the emission seen in our broadband images.”

The results show how Webb’s high angular resolution will allow astronomers to conduct highly accurate infrared observations of large populations of SNRs in galaxies beyond the Magellanic Clouds. This includes M33, the Andromeda Galaxy (M31), and neighboring Local Group galaxies like the Southern Pinwheel Galaxy (M83), the Fireworks Galaxy (NGC 6946), the Whirlpool Galaxy (M51), multiple dwarf galaxies in the Local Group, and many more! Said Sarbadhicary:
“Personally, I am quite excited about being able to study the population of SNRs impacting dense gas with JWST since the physics of how shocks impact dense gas and regulate star formation in galaxies is a major topic in astronomy. The infrared wavelengths have a treasure trove of ionic and molecular lines (like H2 we found) that are excited in warm, high-density gas clouds by shocks, so these observations can be really useful.
“There are also some rare Cassiopeia A-like SNRs in these galaxies that are very young and rich in ejecta material from the explosion, and JWST can provide a lot of new information from emission lines in the infrared. Another big area of study is dust and how they are produced and destroyed in shocks.”
Further Reading: arXiv
Leave a Reply