NOVEMBER 28, 2022 by Ingrid Fadelli, Phys.org
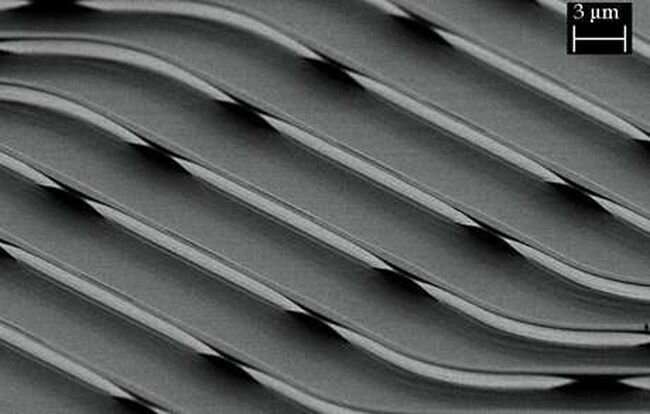
Quantum memory devices can store data as quantum states instead of binary states, as classical computer memories do. While some existing quantum memory technologies have achieved highly promising results, several challenges will need to be overcome before they can be implemented on a large scale.
Researchers at the AWS Center for Quantum Networking and Harvard University have recently developed a promising quantum memory capable of error detection and with a lifetime or coherence time (i.e., the time for which a quantum memory can hold a superposition without collapsing) exceeding 2 seconds. This memory, presented in a paper in Science, could pave the way towards the creation of scalable quantum networks.
Quantum networks are systems that can distribute entangled quantum bits, or qubits, to users who are in different geographic locations. While passing through the networks, qubits are typically encoded as photons (i.e., single particles of light).
“These qubits need to be routed and processed, both to distribute them to different users and to overcome the distance limitations imposed by the transmission loss in fiber optics,” David Levonian, quantum research scientist at AWS, told Phys.org. “A quantum memory is a small quantum computer that can catch and store quantum bits that are encoded in photons without measuring them, as measuring them would destroy any entanglement that they have. The stored quantum bits can be processed and reencoded in photons if necessary.”
So far, physicists and engineers have proposed several different systems that could act as quantum memory that would enable the implementation of quantum networks, such as dilute atomic gases and rare earth ions embedded in glass. The system created by Levonian and his colleagues relies on so-called silicon-vacancy centers (SiVs). These are quantum bits that are made up of electrons around single silicon atoms, embedded in diamond crystals.
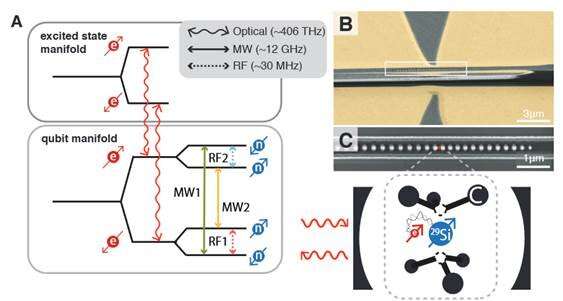
“We build guides for photons that can concentrate them near our SiVs and boost the interaction of light with the electrons,” Levonian said. “At the end of the day, our system resembles the optical modulators that transmit most internet traffic. Like the optical modulators, our quantum memories are switches that either transmit or reflect light depending on whether they’re ‘on’ or ‘off.’ Unlike normal modulators, ours are switched on and off by a single electron, rather than large electrical signals, and can be in a quantum superposition of on and off.”
The researchers’ study had two primary objectives. The first was to explore the possibility of using the magnetic fields of individual nuclei as quantum memories with longer lifetimes (i.e., that can store information for longer). The second was to create a quantum memory that could operate at higher temperatures.
“Our study’s first objective is a common one in our subfield of solid-state quantum memories,” Levonian said. “Basically, electron spins are very convenient for interacting with photons, but also sensitive to magnetic and electric fields. This sensitivity reduces their coherence times, which has motivated researchers to look at ways to transfer quantum information from electrons to more inert nuclear spins. In our paper, we looked at transferring to quantum information to the spin of the silicon atom that forms the SiV.”
To achieve their second objective, that of operating their quantum memory at higher temperatures than those reported so far, the researchers had to devise strategies that would reduce their SiVs’ sensitivity to phonons (i.e., thermal vibrations in the diamond lattice), as phonons can scramble quantum bits encoded in the SiV. Past physics studies predicted that an SiV in a squeezed or “strained” crystal should be less sensitive to phonons.
This prediction was also confirmed by previous experiments, utilizing squeezed SiVs based on unstructured diamond. Levonian and his colleagues wished to reproduce this previously reported effect in their SiV-based quantum memory.
“In the end, we were able to achieve both of our objectives—we were able to catch and store photons at 4 degrees Kelvin, instead of the 0.1 degrees that previous experiments had operated at, and we were able to take the information from photons we had stored on the electron, swap it to the silicon nucleus and store it about 1,000X longer,” Levonian said. “In the field of possible quantum memories, our technology represents a happy medium on many axes.”
The quantum memory created by this team of researchers can “hold on” to a quantum bit for a couple of milliseconds, which is a relatively long coherence time, although not the longest reported in the literature so far. Similarly, it can process local quantum information without errors 99% of the time, which is better than many platforms but worse than the very best.
“Where our memory really shines is in its efficiency at gathering up and storing photons—currently around 50% and soon to improve even more,” Levonian explained. “Another important feature is that it is a ‘heralded’ quantum memory—it gives a signal when it catches a photon. This ends up being crucial for designing efficient quantum networks.”
While the new quantum memory’s ability to operate at 4 degrees Kelvin instead of 0.1 degrees Kelvin (as previously proposed devices) might not seem like a huge step forward, it could have significant consequences for the future large-scale implementations of quantum networks. In fact, cryogenic refrigerators that can bring the temperature down to 4 Kelvin are about 5 times cheaper and 10 times smaller than refrigerators that cool objects down to 0.1K, and can also fit in a server rack.
“Getting to 0.1 Kelvin requires a dilution fridge (the ‘golden chandelier’ that readers might have seen in articles on quantum computing), and the relatively rare and expensive Helium 3 isotope,” Levonian added. “Our success in this study came partly from luck, as we discovered that one of our devices had become quite strained during our manufacturing process, which let us test the temperature resilience this imparted to the SiVs. To use our discovery to build commercial devices, we need to find a way to make strained SiVs 100% of the time, which is something we’re actively working on.”
More information: P.-J. Stas et al, Robust multi-qubit quantum network node with integrated error detection, Science (2022). DOI: 10.1126/science.add9771
Journal information: Science
Leave a Reply